The effect of frequency in welding thick-walled HFI
pipes
Hendrik Loebbe |
Introduction
The use of High Frequency Inductive (HFI) welding is a highly productive process
for the manufacture of longitudinally welded pipes from hot‑rolled strip.
Longitudinally welded HFI pipes are nowadays used in the most diverse range of
applications. Typical of such uses are, for example, pipelines for the
conveyance of liquid and gaseous hydrocarbons, potable and utility water, brine,
district heating systems, hollow sections and Oil Country Tubular Goods (OCTG).
Mannesmann Fuchs Rohr (Siegen and Hamm, Germany) produces HFI pipes in O.D.
4”-20” (114.3-508.0 mm) with wall thicknesses up to t = 0.81” (20.6 mm). Special
requirements originating from the offshore sector are thus also fulfilled via
the use of high‑quality grades of steel with large pipe‑wall thicknesses [1]. In
this sector, HFI pipes are increasingly coming into use in the offshore sector,
in competition with SAW and seamless pipes. Not least important of all in this
context is the trend toward the production of ever thicker‑walled HFI pipes. The
technological preconditions necessary for this purpose, in terms of HFI welding
technology, are discussed, with attention to normative aspects, in the following
essay, with prime emphasis attended to the topic of "welding frequency".
The welding process
The welding process is the central operation in the chain of production
processes involved in the manufacture of pipes from hot‑rolled wide strip. One
can differentiate essentially between fusion welding and pressure welding, with
a number of diverse process variants [2]. Fusion welding methods take the form
of the submerged‑arc and gas metal-arc welding processes for production of
longitudinally welded and spiral‑welded pipes. The pressure welding processes
include among others the low-frequency and the high‑frequency welding, the
latter being the subject of this essay. There are in the relevant literature no
generally applicable differentiations based, in particular, on physical
principles and/or phenomena for definition of the high‑frequency technology
frequency range, particularly in the case of welding. Minimum frequency values
for HF welding can be found in international product standards and
specifications for pipes, such as EN 10208-2, ISO 3183-2, ISO 3183-3 and API
Specification 5L [3],[4],[5],[6]. Here, a frequency of f ≥ 100 kHz is required
for HF‑welded pipes, irrespective of all other boundary conditions (API
Spec. 5L, PSL 2 only). No upper frequency limit is stated, however. Elsewhere,
the term "high‑frequency" is defined, for example, as the "f = 50 to 10,000 kHz"
or "f = 10 to 5,000 kHz" frequency range [7],[8].
It is possible, in principle, to differentiate in the context of HF welding
processes between conductive and inductive transfer of energy. In the case of
conductive technology, input of energy is accomplished with copper contacts via
the surface of the pipe, with the resultant possibility of strikes and burns on
this surface. In inductive systems energy transfer is accomplished without
contact. In both cases, it must essentially be assured that the necessary "skin
effect" is generated. Utilizable minimum frequencies were in the past the result
primarily of the thermionic valve‑based generator technology and the power thus
available. The advance of transistor technology permits the use of lower
frequencies combined with higher power, particularly in the case of the HFI
process. This is useful, in particular, for welding of thick‑walled HFI pipes
which is one factor permitting the increased use of such longitudinally welded
pipes in offshore application. Anyway, the minimum frequency in this context is
100 kHz, in order to fulfill the requirements of the various standards and
specifications.
Physical effects
In both conductive and inductive HF welding, the principle is based on the fact
that a current flows on the pipe surface and the band edges (Fig. 1). This
high‑frequency current flows on the surface of the pipe via the edges of the
strip to the welding point at which the melted strip edges are forced together
and welded.
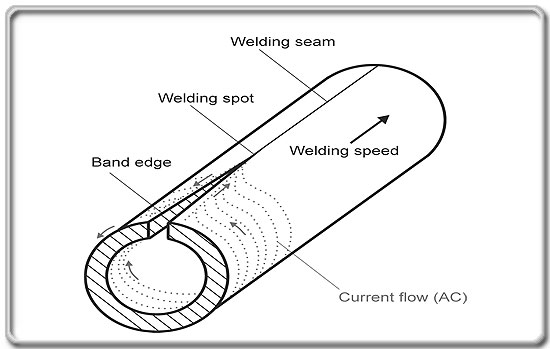 |
Fig. 1: Current flow on the surface of the pipe |
|
The precondition for the achievement of the flow of current on the pipe surface
and on the edges of the strip is the so‑called "skin effect" (Fig. 2). A further
increase in current density on the strip edges results in the "proximity
effect". The current's penetration depth is determined essentially by the
temperature of the material relative to the Curie temperature.
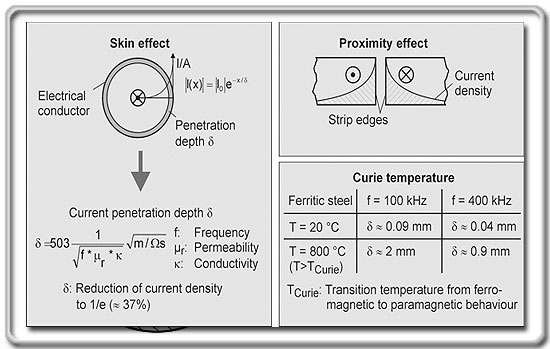 |
Fig. &2: Basic principles [9],[10],[11] |
|
The skin effect causes on the surface of a conductor an increase in current
density dependent on frequency. Current density drops in the form of an
e‑function as distance from the conductor edge increases. Reduction of current
density to 1/e (approx. 37 %) is defined as current penetration depth. The
influencing factors are material‑dependent characteristics and frequency.
Frequency thus provides a method of controlling penetration depth and
temperature increase in the boundary zone of the band edges. It must be noted
that influence is relative to the square root and that a quadrupling of
frequency thus results in a halving of penetration depth. In the case of HFI
welding, the skin effect results in the current flowing only on the pipe surface
and in heating up of the strip edges to welding temperature at the welding
point.
The proximity effect also causes a further increase in current density in the
strip edge zone. This effect is based on the fact that the charges, with
currents flowing in opposite directions, attract each other.
The third aspect with a significant influence on the profile of temperature of
the strip edge is the excess of Curie temperature. This occurs, in accordance
with the iron‑carbon diagram, at T = 769 °C [2]. Ferritic steels exhibit
ferromagnetic behaviour below this temperature, and paramagnetic behaviour above
it. This has a significant effect on the current's penetration depth.
Penetration depth will rise, for example, around 22‑fold if the temperature at
the strip edge rises above the Curie temperature during HF welding.
The dependence of temperature profile on frequency
The heating profile at the edges of the strip can be manipulated, inter alia,
via the selection of frequency. It must be technologically assured that the skin
effect is achieved. The penetration depth of the heat‑affected zone (HAZ),
particularly at the center of the strip, is generally increased as frequency
decreases. The irregular configuration of the heat‑affected zone, in the form of
an hour‑glass, is lessened. This results in the steel's welding temperature also
being reached with processing certainty at the center of the strip (Fig. 3, Fig.
4). The overheated corners of the strip edges possible at higher frequencies are
eliminated at lower ones, such as may be used in HFI process.
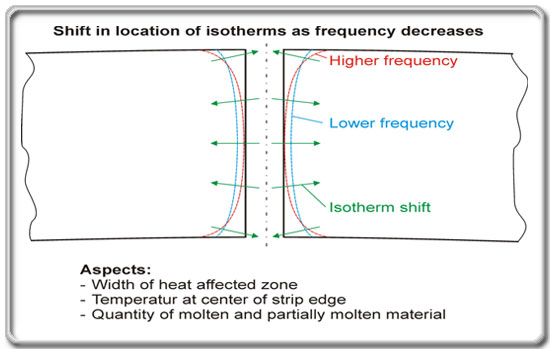 |
Fig. 3: The effects of frequency on temperature profile in
connection with HFI welding |
|
|
Fig. 4 Heating profile at strip edges in production of
thick-walled pipe |
|
Only a few systematic investigations of the influence of frequency on the
process, on the one hand, and on the technological properties of the pipe, on
the other hand, have been published. A number of these are discussed below.
The development of transistor technology has made it possible to use frequencies
below f = 200 kHz for the purpose of HFI welding [12]. In the past, due to
valve‑based technology, the minimum frequency from a technological and economic
standpoint was, on a system‑engineering view, around f = 250 kHz. Extremely fast
MOSFET transistors have been in use in HFI resonant circuits since the
mid‑1980s; in addition, high‑current IGBT transistors have increasingly become
established in the welding‑systems sector in around the last ten years, and thus
permit frequencies down to 100 kHz for HFI welding [12],[13]. Both types of
transistor are nowadays used, depending on their strengths and the corresponding
requirements. The potential for technologically and economically rational use of
frequencies down to 100 kHz for HFI welding must, as already mentioned above,
also be viewed in close conjunction with the trend toward production of ever
thicker HFI pipe‑wall thicknesses.
Finite Element Method (FEM) analysis studies have been performed into the
influence of various process parameters, and frequency, in particular, on the
HFI welding process [14],[15],[16]. The following influencing factors were
examined here: welding angles, distance of the welding point from the inductor,
resilient flexing of the strip edge, welding speed and welding frequency. The
reasons for performance of these published investigations can be found in
welding problems encountered in the production of pipes with a wall thickness of
t = 12.7 mm, which were attributed to a cold strip center. It was ascertained
that all the factors investigated significantly influence the configuration of
the heat profile in the edge of the strip. As far as frequency is concerned, the
frequency 100 kHz cause a much more uniform increase in temperature up into the
center of the strip edge than the frequency 300 kHz. The percentage of melted
material in the vicinity of the corners of the strip edge falls significantly as
frequency falls, thus reducing burn‑off of these corners. It becomes apparent in
the relevant investigations, however, that the other parameters examined also
have a significant influence on the heat profile in the edge of the strip
material and must therefore also be used for description of the process. A
factor of two in the case of the angle of approach of the weld vee, for example,
thus has a greater influence than a factor of three for frequency.
In contrast to this, there are also studies concerning the influence of
frequency, the essential criteria of which are the spread of the heat‑affected
zone and thus necessary power [17],[18]. These investigations are also unanimous
in stating that penetration depth in the f = 100 to 600 kHz range becomes
greater as frequency falls. The unbalanced conclusion of these authors, failing
to take account of other technological boundary conditions, is in this case that
higher frequencies are assumed to be more economical, since a greater
penetration depth would be a waste of energy, and that higher power is therefore
necessary. With respect to the complete melting of the edges of the strip, this
must be critically evaluated, however. At lower frequencies in the case of HFI
welding, heating up to the center of the strip is assured with processing
certainty even in the case of greater wall thicknesses.
Practice also demonstrates that the process as a whole, including the
environment in which it takes place, must also be studied, in addition to these
physical principles. If the process chain, including upstream and downstream
facilities, is thus examined, it will be necessary, for example, to analyze the
interaction of possible power input and welding speed. The principal objective,
that of producing, cost‑effectively, pipes which meet the specification, must
continuously be borne in mind during all such deliberations, however.
The use of adjustable‑frequency HF generators is recommended for large
wall‑thickness/diameter ratios [19]. A significant advantage is, accordingly,
the use of a frequency tailored to the particular application, in order to
minimize the hour glass and therefore the "waist‑like" constriction of the HAZ,
and thus create a very homogeneous weld, with a uniform hardness distribution.
The author does not state exemplary frequencies for specific applications,
however.
All in all, these investigations indicate that the beneficial effects of a lower
frequency on the HFI welding process are clear. There are, on the one hand, only
few well founded publications on this subject and, on the other hand, diverse
conceptual interpretations, however. Practice at Mannesmann Fuchs Rohr indicates
that a uniform heat‑affected zone and extremely good welding results are
achieved. Uniform melting of the entire edge of the strip is assured,
particularly in production of pipes with large wall thicknesses of up to
t = 20.6 mm.
Control of heat input to the edges of the strip
As already outlined above, heat input and the heat profile in the edge of the
strip in HFI welding depend not only on the frequency used, but also on a large
number of other influencing factors. Major influencing factors are shown in Fig.
5. These can be subdivided into six groups, i.e., ambient media, energy
conversion at the inductor, strip‑edge condition, impeder state and arrangement,
welding speed and welding vee. A total of sixteen different parameters, of which
current frequency is one, are shown here. Frequency is, without doubt, an
important factor in control of the welding process and thus the welding result,
but can only be examined in context, together with the other parameters. A
number of results from [14],[15],[16] on this subject have already been
discussed. Detailed examination of the influence of each individual factor is
not the subject of this essay.
|
Fig. 5: Factors influencing heat input
|
|
The frequency and power of HFI systems
Two types of transistor are in general use in HFI welding systems at present
[12],[13],[20]. "Metal Oxide Semiconductor Field Effect Transistors" (MOSFET)
are used in the f > 150 kHz range. "Insulated Gate Bipolar Transistors" (IGBT)
make it possible to provide power of adequate magnitude for the welding of pipes
even at f < 150 kHz.
The spectrum of available frequencies and powers for HFI systems is shown on the
basis of system manufacturers information in Fig. 6. Both technological,
economic and normative aspects must be taken into account in selection of
frequency (new investments, order‑specific). In the case of a new investment, in
particular, the entire plant structure, including upstream and downstream
facilities, must also be taken into account.
|
Fig. 6: HFI systems: Frequency and power
([21],[22],[23],[24],[25] in 2006)
|
|
Summary
The further development of transistors and their use in the field of HFI welding
have resulted in the past approximately ten years in significantly broadened
potentials in plant and system‑engineering terms, particularly with respect to
the utilizable frequencies. On the product side, on the other hand, the trend
toward thicker‑walled HFI pipes, which are increasingly coming into use in the
offshore sector, has continued. At present, and less for technological than for
normative reasons, the frequencies used are subject to specific minimal values.
Such process‑engineering restrictions in product‑specific standards should,
however, be consigned to history, and thus no longer stand in the way of
system‑engineering progress. Prime emphasis in this context should be directed
solely at the product properties of the pipes for the particular application. It
must, in general, be noted that HFI welding cannot be defined in terms of
"frequency" as the sole influencing factor. Rather, the interaction of a large
range of factors is of great importance from a production‑engineering viewpoint.
References
- Zimmermann, B.; Brauer, H.; Marewski, U.: Development of HFIW line pipe
for offshore applications. “4th International Conference on Pipeline
Technology”, 9.‑13.05.2004, Ostende, Belgium
- Sommer, B.: Stahlrohr Handbuch. 12th edition, Vulkan‑Verlag Essen, 1995
- N. N.: Technical delivery conditions for steel pipes for use with
combustible fluids. EN 10208‑2, August 1996
- N. N.: Petroleum and natural gas industries – Steel pipe for pipe lines
– Technical delivery conditions. ISO 3183‑3, April 199
- N. N.: Specification for Line Pipe. API Specification 5L, 43rd Edition,
March 2004
- N. N.: Specification for Line Pipe. API Specification 5L, 43rd Edition,
March 2004
- DIN 48600: Industrielle Elektrowärmeeinrichtungen – Leistungsmessung an
Hochfrequenzgeräten für Induktionserwärmungseinrichtungen ‑ Messverfahren
zur Bestimmung der Ausgangsleistung von HF‑Generatoren. February, 1979
- Beitz, W.; Küttner, K.‑H.: Dubbel – Taschenbuch für den Maschinenbau.
18th edition, Springer‑Verlag, 1995
- Schwetje, T.: Untersuchungen zum Hochfrequenzschweißen von
Konturbauteilen. Dissertation, TU Clausthal, 2002
- Dilthey, U.: Materialsammlung zur Vorlesung Schweißtechnische
Fertigungsverfahren I “Schweiß‑ und Schneidtechnologien”. ISF RWTH Aachen (www.isf‑aachen.de)
- Matthes, H. G.; Jürgens, R.: HF‑Rohrschweißen mit IGBT‑Reihenschwingkreisumrichter.
iew – Industrielle Elektrowärme, 56 (1998) 4
- Leistungsschalter für Schweißinverter – Thyristor, BJT, FET und IGBT.
Kemppi GmbH (www.kemppi.com)
- Asperheim, J. I.; Grande, B.; Markegård, L.; Buser, J. E.; Lombard, P.:
Computation and analysis of temperature distribution in the cross‑section of
the weld Vee. Tube Int., Nov. 1998
- Asperheim, J. I.; Grande, B.: Temperature Evaluation of Weld Vee
Geometry and Performance. Tube Int., Oct. 2000
- Grande, B.; Asperheim, J. I.: Factors Influencing Heavy Wall Tube
Welding. Tube & Pipe Technology, March/April 2003
- Scott, P.: The Effects of Frequency in High Frequency Welding.
Transactions of Tube 2000, Toronto, ITA Publication (www.tubenet.org.uk)
- Morin, T.; Scott, P.: Modern Methods of High Frequency Welding Used to
Produce Conistent Quality.
www.tubenet.org.uk
- Gardiner, D.: Variable Frequency on Demand – The Ultimate in Flexibility
for Today’s Tube and Pipe Producers. Tube & Pipe Technology, July 2003
- Quaglia, A.: Selecting the right H.F. Generator.
www.tubenet.org.uk
-
www.sms‑elotherm.com
-
www.emmedi.it
- www.thermatool‑europe.com
-
www.efdinduction‑usa.com
-
www.efd‑induction.com
Contact Details
Mannesmann Fuchs Rohr GmbH
Kissinger Weg
D-59067 Hamm
Germany
Email.
Website:
www.mannesmann-fuchs.com
|